Study shows what stresses Pittwater's seagrass meadows (and the fish that love this estuary habitat) + Jetty design review to protect seagrass
The more human disturbances, the greater the loss of seagrass.
That was the finding of a recent study conducted in the Pittwater estuary by DPI Fisheries.
The study looked at how Posidonia australis seagrass (or strap weed) loss related to the cumulative effects of human impacts such as foreshore development, poor water quality, vessel traffic and prop scour, and anchor damage.
It found that human disturbances are impacting seagrass beds with consequences for fish productivity and biodiversity.
DPI Fisheries Research Scientist Dr Matt Rees led the study.
He said the study was important as it will help protect Posidonia seagrass beds in the future.
“Preserving Posidonia is just so important for the health and ecology of NSW estuaries like Pittwater. Posidonia provides many benefits, one being acting as a critical nursery habitat for many fish species.”
“In Pittwater, like many estuaries across NSW, there has been significant losses of seagrass species like Posidonia, with populations endangered in most of the major Sydney estuaries.”
“We knew seagrass can be lost due to things like shading from poor jetty design, anchoring from boating and poor water quality. What we wanted to better understand was what the cumulative impact of these factors on seagrass is. This paper helps answer that question,” he said.
“Understanding how these factors combine to impact seagrass will help managers make better decisions to reduce future losses,” he said.
The study mapped the distribution and intensity of human impacts across Pittwater. When this map was compared to areas of mapped seagrass across the estuary, the pattern was clear.
“Areas exposed to high human impacts had the greatest losses of Posidonia seagrass over time,” Dr Rees said.
But it is how this loss of seagrass was impacting fish numbers that local fishers might find most interesting.
To determine this, Dr Rees said his team used baited underwater cameras to survey fish across the estuary.
“We found Posidonia seagrass had a unique assemblage of fishes compared to nearby sand habitat,” he said.
“The number of popular target species such as snapper, tarwhine and bream were highest on the edge of seagrass meadows,” Dr Rees said.
“Ultimately, this study shows that it's important to consider how human impacts combine to impact seagrass beds and this has flow on effects to fish biodiversity and fisheries production.”
“We are hoping these research findings will help managers with their decision-making regarding human activities in NSW estuaries to better protect seagrass and fish into the future.”
This study is part of the Threats to estuarine fish assemblages project which is funded via the Marine Estate Management Strategy.
You can find out more about why seagrass is so important on the DPI Fisheries webpage here.
You can read the full paper Cumulative effects of multiple stressors impact an endangered seagrass population and fish communities here.
And runs in full below, under a Creative Commons Licence.
Since this study was published at the end of 2023:
Tackling Climate Change: Estuarine Seagrass Under Stress
September 2024
As climate change progresses, coastal areas of NSW are likely to experience more frequent and intense rainfall.
This increase in freshwater runoff into estuaries poses a serious challenge to aquatic plants like the endangered seagrass, Posidonia australis.
Known for maintaining healthy estuarine ecosystems and providing vital habitats for marine life, Posidonia may now be under greater threat.
Floods can reduce salinity and make estuarine water murkier, making it difficult for Posidonia to photosynthesise.
Recent research led by Dr Tim Glasby, Principal Research Scientist at the Port Stephens Fisheries Institute, aimed to understand how well this seagrass species can withstand these stressful conditions.
He said by simulating extremely low salinity and reduced light over several months, the team observed Posidonia's response to repeated flood events.
"Initial findings reveal that after a single simulated flood, Posidonia lost a significant number of leaves and shoots, affecting its ability to photosynthesise," Dr Glasby said.
However, there was encouraging news: plants that survived one flood were better able to endure subsequent floods.
“The rhizomes and roots of Posidonia remained in reasonable condition even after enduring three simulated floods, suggesting potential for regrowth," he said.
Dr Glasby said this resilience is promising, given Posidonia's important role in keeping our estuaries healthy.
The next phase of the project will explore the resilience of Posidonia seedlings and investigate genetic differences among estuaries to understand how this species adapts to extreme environmental conditions.
"With ongoing research, we hope to secure the future of Posidonia australis, ensuring it continues to support biodiversity in our estuaries even as our climate changes," Dr Glasby added.
This project, ‘Impacts of future climate on the threatened seagrass Posidonia australis’ supports the delivery of the Marine Estate Management Strategy Initiative 3 ‘Estuarine habitat monitoring and threat assessment’. It is primarily funded by the NSW Environment Trust.
Reviewing jetty design to better protect seagrass
On June 26 2024 the NSW Marine Estate released information about its project of Reviewing jetty designs.
Why is this project important?
Jetties and pontoons provide access to the benefits of the marine estate, however poor design can pose a threat to the health of seagrass beds as they reduce the amount of available sunlight.
Seagrass beds are a key estuarine habitat. They provide food and shelter for many marine animals, including commercially and recreationally important fish species. Seagrasses are marine plants and require sunlight to live and grow.
Seagrass meadows also oxygenate water, stabilise sand, improve water quality by recycling nutrients and act as a highly efficient carbon sink.
This project is researching the design features of jetties to determine what leads to the loss of seagrass and develop jetty design guidelines to help reduce this loss.
We are surveying seagrass under and adjacent to jetties across many NSW estuaries to determine what design features lead to seagrass loss. Design features being assessed include the height, width, orientation and construction material of the jetty.
Results from this project will help inform design guidelines to better protect seagrass.
You can learn more about the importance of seagrass in NSW on the Department of Primary Industries and Regional Development Fisheries webpage.
What have they achieved so far?
Over 300 jetties have been surveyed in Port Hacking for seagrass occurrence.
90 diving surveys have been conducted in Port Hacking to measure seagrass biomass under and adjacent to jetties.
''We will be using this information to establish the best practice with the construction of jetties across NSW.'' the Department stated
Lead agency: DPIRD Fisheries
Locations: Estuaries in the Greater Sydney region, Local government areas - Statewide
Example Pic of a design that allows light through: NSW Marine Estate
Out Studying The Tidal Zones And Sea Grass Areas Around Pittwater: summer (January) 2019
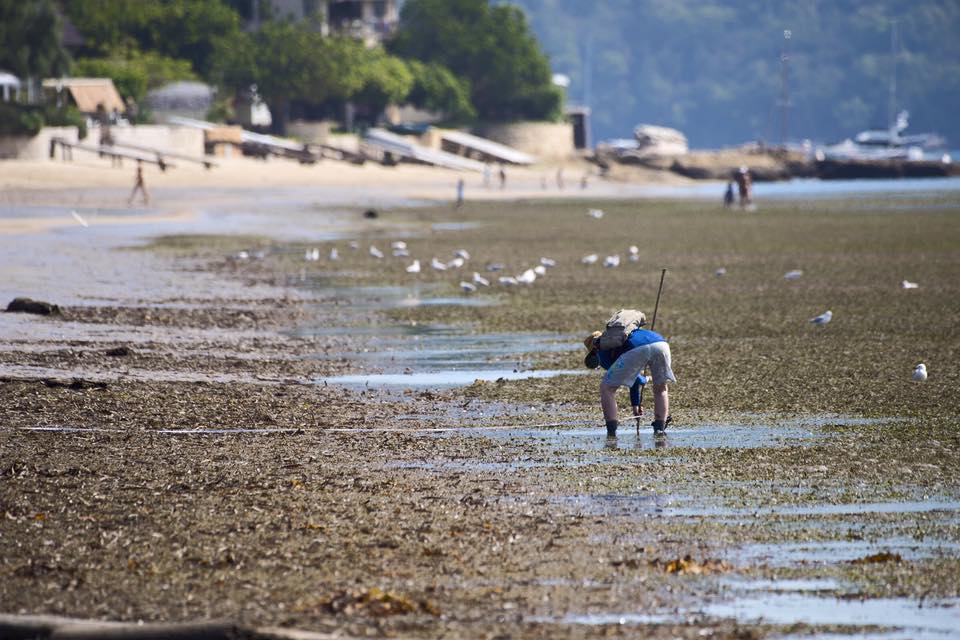
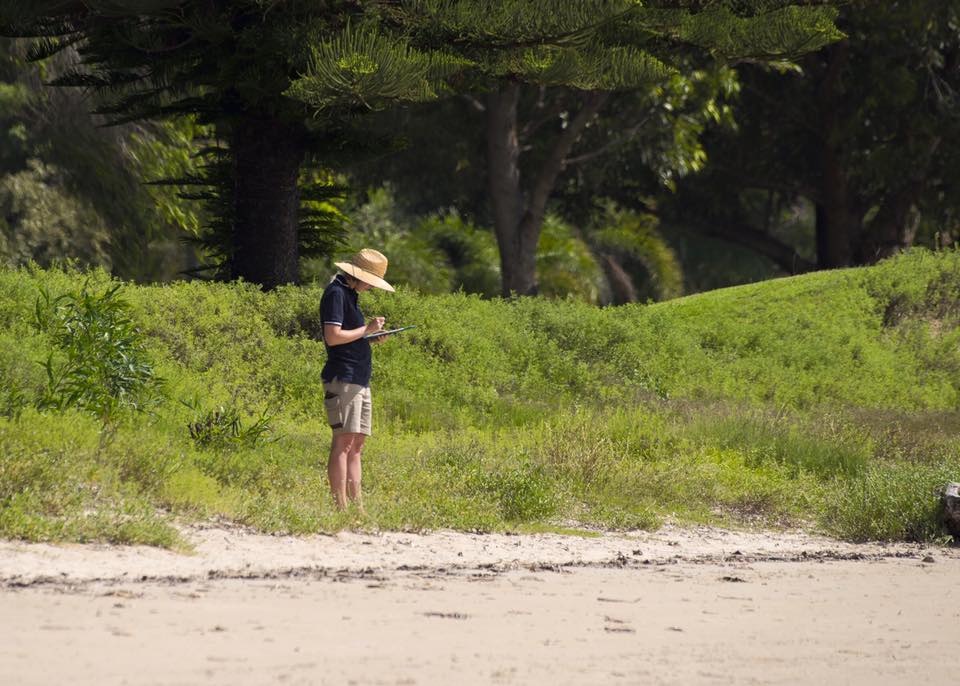
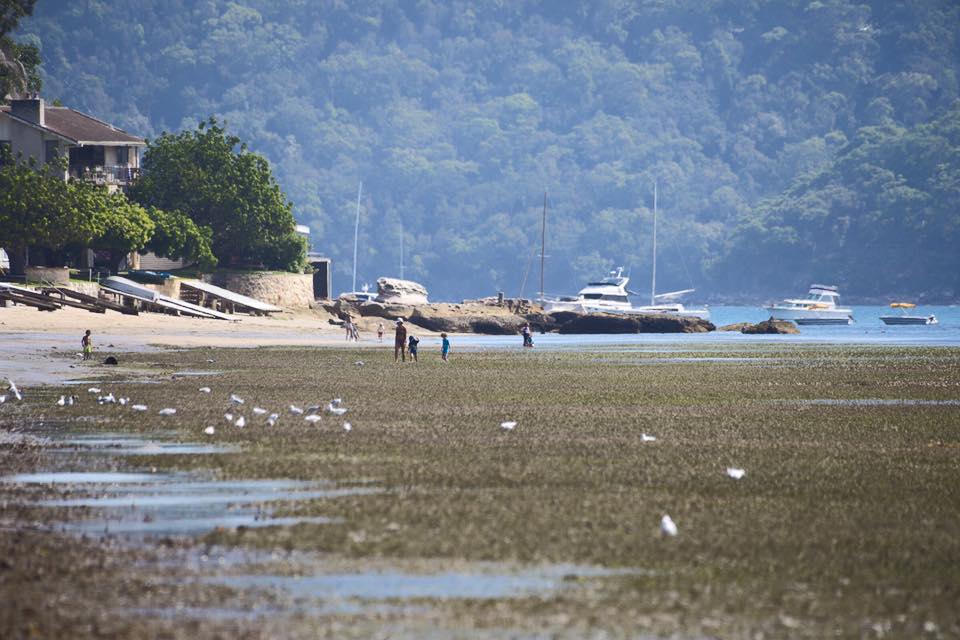
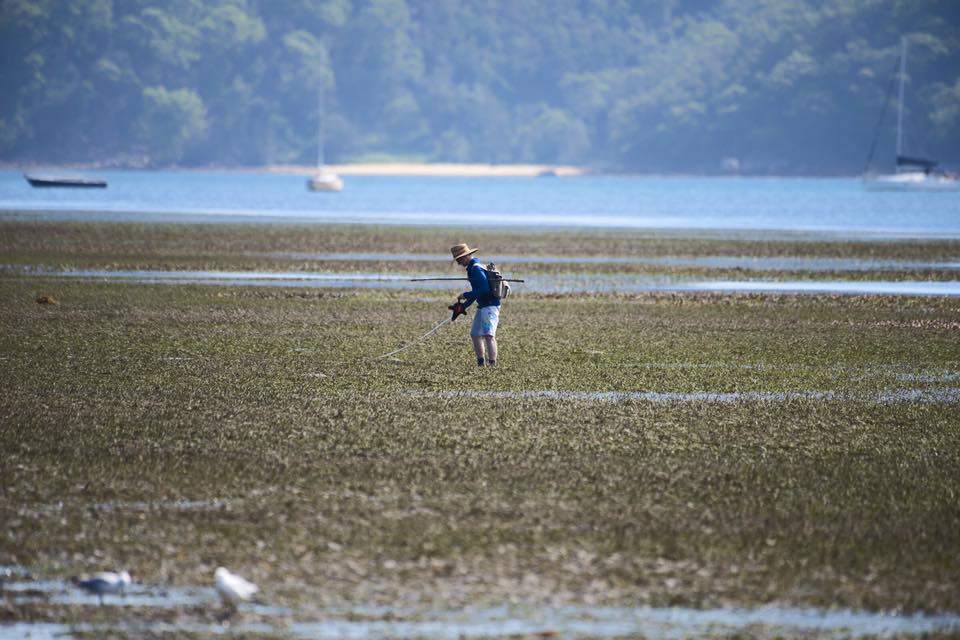
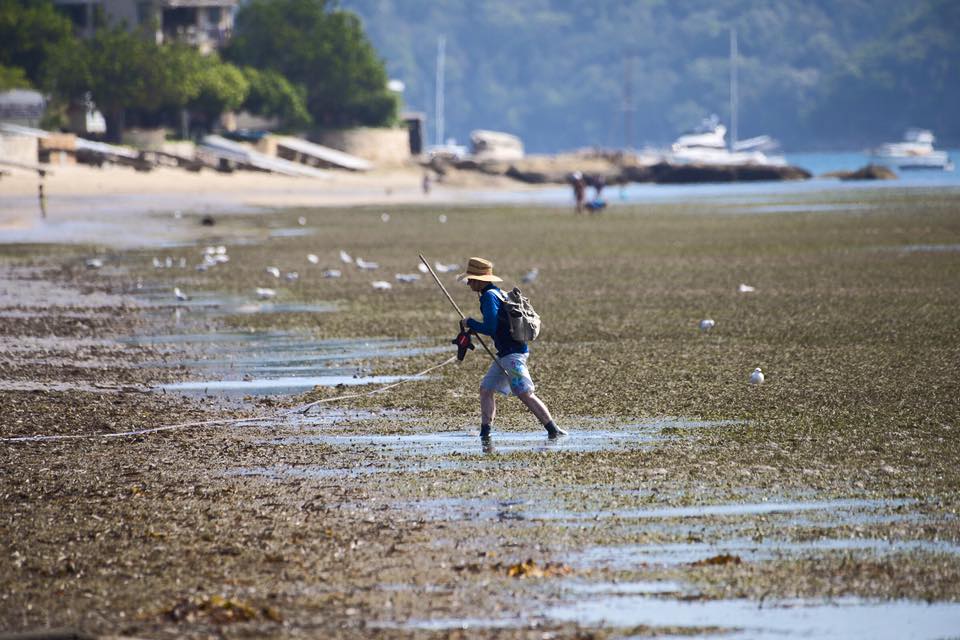
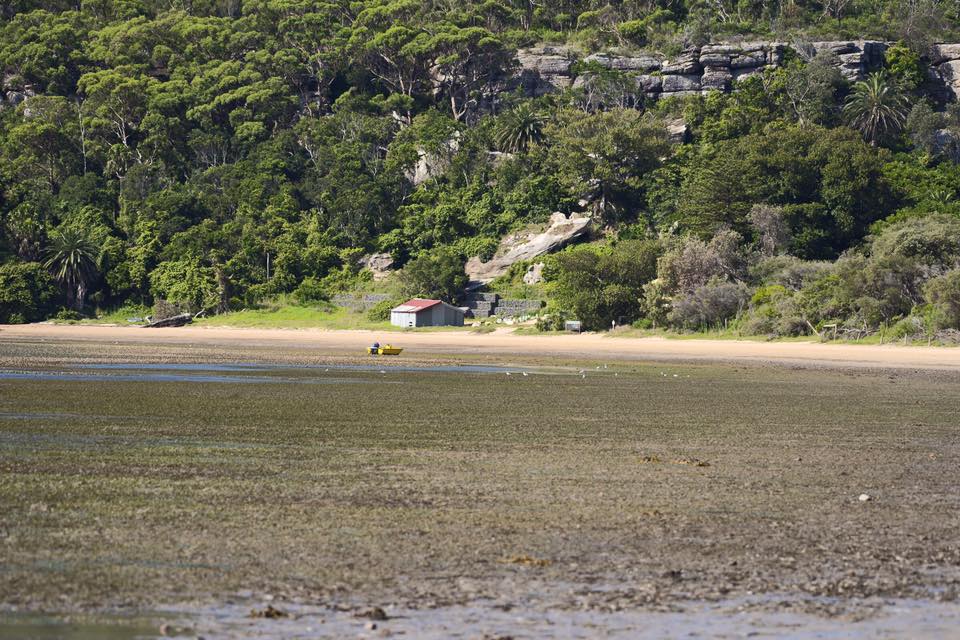
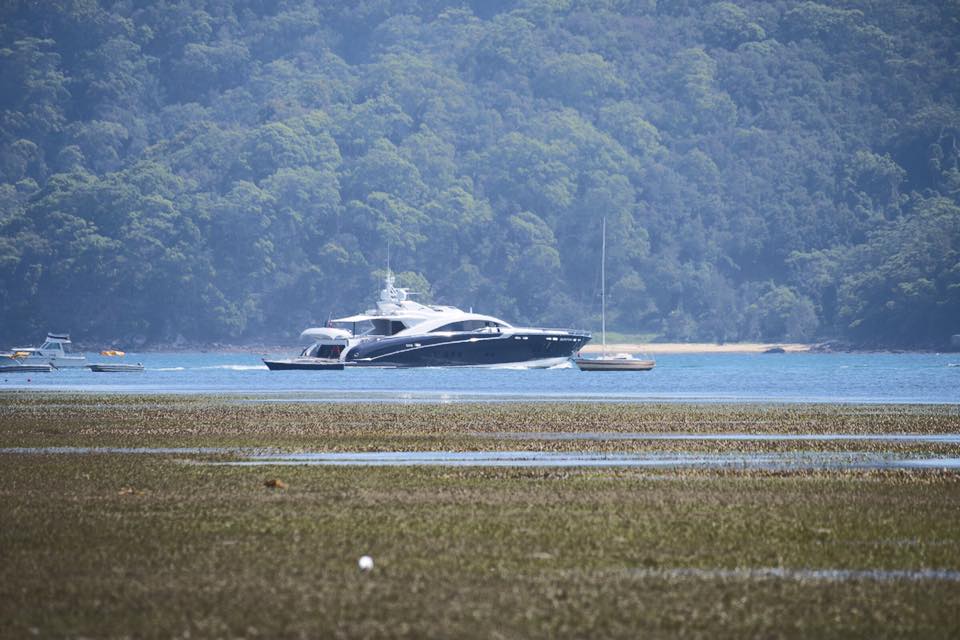
Cumulative effects of multiple stressors impact an endangered seagrass population and fish communities
Abstract:
Coastal ecosystems are becoming increasingly threatened by human activities and there is growing appreciation that management must consider the impacts of multiple stressors. Cumulative effects assessments (CEAs) have become a popular tool for identifying the distribution and intensity of multiple human stressors in coastal ecosystems. Few studies, however, have demonstrated strong correlations between CEAs and change in ecosystem condition, questioning its management use. Here, we apply a CEA to the endangered seagrass Posidonia australis in Pittwater, NSW, Australia, using spatial data on known stressors to seagrass related to foreshore development, water quality, vessel traffic and fishing. We tested how well cumulative effects scores explained changes in P. australis extent measured between 2005 and 2019 using high-resolution aerial imagery. A negative correlation between P. australis and estimated cumulative effects scores was observed (R2 = 22 %), and we identified a threshold of cumulative effects above which losses of P. australis became more likely. Using baited remote underwater video, we surveyed fishes over P. australis and non-vegetated sediments to infer and quantify how impacts of cumulative effects to P. australis extent would flow on to fish assemblages. P. australis contained a distinct assemblage of fish, and on non-vegetated sediments the abundance of sparids, which are of importance to fisheries, increased with closer proximity to P. australis. Our results demonstrate the negative impact of multiple stressors on P. australis and the consequences for fish biodiversity and fisheries production across much of the estuary. Management actions aimed at reducing or limiting cumulative effects to low and moderate levels will help conserve P. australis and its associated fish biodiversity and productivity.
1. Introduction
Globally, the human population is disproportionally concentrated along coastlines (Barragán and de Andrés, 2015) exposing coastal ecosystems to a variety of human activities and associated stressors including pollution (e.g. nutrients and contaminants), fishing (biomass removal), physical disturbance and non-indigenous species invasion (Dafforn et al., 2015;Diaz and Rosenberg, 2008; Jackson et al., 2001; Knott et al., 2009; Lotze et al., 2006). In addition, climate change may disproportionately affect coastal ecosystems due to increased likelihood of storm events and marine heat waves (He and Silliman, 2019). There is increasing awareness that stressors in coastal zones are unlikely to operate in isolation (Crain et al., 2008; Simeoni et al., 2023) and that stressor co-occurrence may have profound effects on marine organisms and communities (Przeslawski et al., 2015). The consequences of multiple stressors – termed cumulative effects – may lead to complex changes to ecosystems condition (Griffith et al., 2012). Given the increasing exposure of coastal environments to human activities and associated stressors, cumulative effects represent a knowledge gap for the management of coastal ecosystems (Fleishman et al., 2011; Griffith et al., 2020; Halpern et al., 2019).
Cumulative effects assessments (CEAs) have become a popular method to estimate the effects of multiple stressors to coastal ecosystems (Halpern and Fujita, 2013) and have strong potential to guide management responses (Allan et al., 2013; Anthony et al., 2013; Stelzenmüller et al., 2018). The most common approach in the literature, advanced by Halpern et al. (2008), combines multiple stressors into a single comparable estimate of cumulative effects. This is achieved by mapping the spatial distribution and intensity of each stressor or human activity in the study region across the ecosystem or species of interest. Impact weights of each stressor to a particular ecosystems or species are determined by expert judgement and are summed to generate the cumulative effect scores. Given the availability of spatial data on the distribution and intensity of stressors compared to data on the condition of a species, community or ecosystem, CEAs are becoming increasingly used, particularly in guiding management decisions in coastal settings (Hammar et al., 2020; Stelzenmüller et al., 2020; Tulloch et al., 2020).
For CEAs to be useful for coastal management, cumulative effects scores must adequately represent the condition of a habitat, community or ecosystem in relation to multiple stressors (Halpern and Fujita, 2013). Despite the popularity and intrinsic value of this approach, this key assumption is rarely tested with empirical data (but see Clark et al., 2016; Rullens et al., 2022; Stockbridge et al., 2021). This undoubtedly is due to the challenges and costs of monitoring ecosystem condition at appropriate scales and the difficulties in identifying appropriate measures of condition. Without an understanding of the performance of cumulative effects scores in predicting ecosystem condition there is a high likelihood that CEAs may be providing misleading conclusions (Stockbridge et al., 2021). This is particularly problematic for resource managers and scientists who use CEAs to guide their decision-making.
Despite a few recent examples of CEAs being applied to seagrasses, to our knowledge only one study has explicitly assessed whether seagrass condition correlates with cumulative effects scores. In the Spencer Gulf, South Australia, seagrass condition measures (using indices incorporating percentage cover, area, patchiness, connectivity, and species diversity) estimated from towed underwater video were not strongly associated with cumulative effects scores. However, two genera (Zostera and Halophila) included in the seagrass condition measures from the Spencer Gulf can fluctuate considerably in density and area through time (Kilminster et al., 2015; West and Glasby, 2022) meaning that static condition measures of these genera may not be sensitive to impacts of cumulative effects. Applying a CEA to only enduring species with limited natural fluctuation in extent may provide a more sensitive test of whether cumulative anthropogenic disturbances correlate with reductions in seagrass area. For persistent seagrasses that typically form enduring meadows, measuring changes in its spatial extent using high-resolution aerial photography and satellite imagery (Rolfsema et al., 2014; Swadling et al., 2023; West and Glasby, 2022) provides an opportunity to validate CEAs for these species in a cost-effective manner. This also enables the entire seagrass population to be sampled, meaning that the full scale of potential stressors within the estuary can be assessed.
Seagrass meadows are important habitats prevalent in nearshore coastal ecosystems and a significant focus for management as they are susceptible and threatened by a range of human activities (McMahon et al., 2022; Orth et al., 2006; Unsworth et al., 2019a). Seagrasses provide a range of ecosystem functions and services, such as primary production (Duarte and Chiscano, 1999), sequestration of carbon (Gaylard et al., 2020), nutrient cycling (Stapel et al., 1996) and protection of coastlines from erosion (Christianen et al., 2013; Ondiviela et al., 2014). Notably, seagrasses are also considered an essential habitat and nursery area for numerous fish species of high recreational, commercial and cultural significance, which benefit from the high availability of food and shelter (Beck et al., 2001; Gillanders, 2006). Seagrasses are experiencing global widespread loss and fragmentation due to multiple anthropogenic disturbances, with an estimated 19.1 % of surveyed meadow area lost since 1880 (Dunic et al., 2021). Declines in seagrass coverage have been attributed to a variety of human activities such as land-use changes, overdevelopment, boating infrastructure, aquaculture and fishing (BMT WBM, 2017; Broad et al., 2020; Glasby and West, 2018; Howarth et al., 2022; Orth et al., 2006; Swadling et al., 2023; Turschwell et al., 2021). Such activities generate stressors to seagrass such as increased turbidity, eutrophication, physical destruction, and shading (Ostrowski et al., 2021; Stockbridge et al., 2020). As a result, CEAs can determine areas where seagrass is at greatest risk from cumulative effects, which in turn can guide or optimise management actions (Brown et al., 2014; Clark et al., 2016; Giakoumi et al., 2015; McMahon et al., 2022; Murphy et al., 2022).
In this study we develop a map of cumulative effects from multiple human activities and associated stressors to the persistent seagrass Posidonia australis in a highly urbanised estuary where the species is listed as endangered in NSW, Australia. Specifically, we assessed whether there was a relationship between cumulative effects scores generated from multiple anthropogenic activities and stressors (foreshore development, water quality, commercial vessels, and fishing) to P. australis condition measured as the change in area between 2005 and 2019. As human activities and stressors are more concentrated at shallower depths and along shorelines, depth was also included as a predictor of P. australis condition. Given that a key ecosystem service provided by seagrass is habitat for fish, further aims of our study were to assess 1) the flow-on effects of cumulative impacts to P. australis on estuarine fish biodiversity and 2) the abundance and length of sparids (Acanthopagrus australis, Chrysophrys auratus and Rhabdosargus sarba) relative to contemporary P. australis cover. Sparids were examined as they were the most abundant fisheries species observed in the assemblage. Here, we predicted that P. australis would contain a distinct assemblage of fishes compared to non-vegetated sediments, and that on non-vegetated sediments, distance to P. australis would be an important predictor of the abundance and size of sparids. Understanding these patterns would allow estimation of the wider flow-on effects of multiple stressors to P. australis.
2. Materials and methods
2.1. Study area
This study was completed in the Pittwater estuary (33.6291°S, 151.3016°E), which is located 30 km north of Sydney and is a tide-dominated, drowned river valley arm of the Hawkesbury-River estuary (Roy et al., 2001) covering an area of approximately 18 km2. Pittwater has relatively low freshwater inputs and high marine inputs from the open ocean, so the salinity and water temperature are relatively uniform throughout the estuary (Bell et al., 1988). The Pittwater catchment (approximately 51 km2) is highly urbanised along its southern and eastern shore with some light industry in the southern catchment (Birch et al., 2012). In contrast, the western shoreline borders the Ku-ring-gai National Park with low levels of urbanisation and no industry land-use (WBM Oceanics Australia, 2006). Given the gradients in urbanisation, Pittwater represents a highly suitable estuary to assess the effects of multiple human stressors on environmental assets, such as the seagrass Posidonia australis.
2.2. Posidonia australis
Posidonia australis Hook.f. is endemic to temperate Australia and inhabits sheltered marine and estuarine waters. It is a persistent species that forms extensive enduring meadows with relatively low temporal variability in extent (West and Glasby, 2022). P. australis has limited potential to recover from anthropogenic impacts due to slow growth rates and, in NSW, low fecundity (Larkum, 1976; Meehan and West, 2000). Small-scale losses of P. australis may take decades to recover naturally (Meehan and West, 2000). Indeed, P. australis has suffered historical losses across much of its distribution in Australia, most notably in heavily urbanised estuaries (Cambridge et al., 1986; Larkum and West, 1990; West and Glasby, 2022). The loss and degradation of P. australis meadows has led to the species being listed as endangered in six NSW estuaries under state legislation (NSW Fisheries Management Act 1994) and as a threatened ecological community in nine estuaries (including the six in state legislation) under national legislation (Environment Protection and Biodiversity Conservation Act 1999). Pittwater is one of the estuaries where P. australis is an endangered population and threatened ecological community. The life-history traits of P. australis and its high conservation significance make it a good candidate to assess relationships between areal change and cumulative effects, as any decreases in its area likely relate to human disturbances rather than natural fluctuations and the results will be important for management.
2.3. Mapping of Posidonia australis
Meadows of Posidonia australis in Pittwater were mapped from aerial imagery in 2005 and 2019. Seagrass maps from 2005 were created using a digital GIS approach where P. australis meadows were manually digitised using onscreen digitising techniques at a scale of 1:1500 using the best available ortho-rectified imagery. In comparison, the 2019 mapping was completed using Object Based Image Analysis (OBIA) techniques using Trimble eCognition™. This technique generates the initial seagrass polygon boundaries by segmenting high-resolution (0.3 m) ortho-rectified imagery into image objects based on similar colour, texture and shape. The derived image objects were then manually classified to identify seagrass species. Each mapping method produced initial presumptive maps that were field validated using a combination of high accuracy differential GPS, towed underwater videos, drones (in 2019) and side-scan sonar. These presumptive maps were then updated using field observations to create final mapped products. Both mapping methods produced highly detailed maps suitable to investigate change through time (West and Glasby, 2022). In this study any polygons that contained P. australis were classified as Posidonia (West and Glasby, 2022). Seagrass maps used in this project can be viewed on the NSW Estuarine Habitat Dashboard (NSW DPI, 2021). We used total change in P. australis extent (m2) between 2005 and 2019 as a metric for ecosystem condition. Change in extent was calculated across a 100 m by 100 m fishnet grid of square polygons throughout Pittwater where P. australis occurred in at least one of the sampling years. The grid of P. australis change in cover consisted of 553 cells throughout the estuary, which was calculated using ArcGIS v10.6.1.
2.4. Mapping human activities/stressors
High-risk human activities/stressors to seagrass were mapped across the gridded extent of Posidonia australis. These were identified using a qualitative ecological risk assessment of key habitats in Pittwater (Astles et al., 2015; Table S1). High risks to seagrass included foreshore development, stormwater and catchment run-off, commercial vessels and fishing (as described and justified in Table S1). In total, 11 stressors were measured across the estuary for the high risk activities, namely 1) the density of private and public boat moorings, 2) the total area of jetties, pontoons and marinas combined, 3) the total area of seawall, 4) distance to stormwater outlet, 5) lead sediment concentration, 6) zinc sediment concentration, 7) copper sediment concentration, 8) the presence or absence of ferry terminals, 9) boat-based recreational fishing, 10) shore-based recreational fishing and 11) commercial fishing. All layers were calculated across the 100 m by 100 m grid. The area of jetties, pontoons, marinas and seawalls were mapped using OBIA techniques. These layers can be viewed on the Estuarine Habitat Dashboard (NSW DPI, 2021). Distance to a stormwater outlet was calculated using Euclidian distance and total heavy metal concentrations (Pb, Zn and Cu) in sediment were obtained from Birch et al. (2012). The heavy metal concentration data were extrapolated across Pittwater using ordinary kriging analysis in ArcGIS following Birch et al. (2012) and extracted to each grid. The commercial vessel layer was the presence, or absence of a commercial ferry wharf or berth in a cell. Data on boat-based and shore-based recreational fishing intensity was generated from recreational fishing survey data (Steffe and Murphy, 2011) and extrapolated across the Pittwater estuary using kernel density estimates in ArcGIS. Mean values for boat-based and shore-based fishing were calculated for each cell. The commercial fishing layer was whether the area was open, or closed to commercial fishing under the NSW Fisheries Management Act, 1994.
2.5. Mapping cumulative effects
To generate cumulative effects scores and a cumulative effects map for Posidonia australis in Pittwater, we followed the general framework developed by Halpern et al. (2008). First, we log-transformed and normalized (scaled between 0 and 1) all stressor data. No weightings were applied to the stressors as we do not have sufficient information on the relative importance of all stressors. Expert elicitation could be used to develop stressor weightings; however this process can be affected by biases and uncertainty (Burgman et al., 2011) unless a large number of experts are surveyed and there are specific questions to gauge expert's uncertainty (Jones et al., 2018; Speirs-Bridge et al., 2010). For these reasons we decided to perform the assessment with no stressor weightings. Therefore, stressors ranged between values of 0 (not present in the grid cell) and 1 (the highest density/intensity observed). The exception was the commercial vessel and commercial fishing layers, which were the presence (1) or absence (0) of the activity across the grid. All stressor values were summed across all cells to develop a cumulative effects raster layer for Pittwater. Therefore, the theoretical range of possible cumulative effects scores was 0 to 11, where a score of 11 would indicate a cell with all stressors present at its highest intensity.
2.6. Fish sampling
To survey fish assemblages across Posidonia australis and non-vegetated sediment habitats of Pittwater, a spatially balanced sampling design was created using the ‘MBHdesign’ package in R (Foster, 2021) following national survey design best practices (Foster et al., 2020). Survey sites were generated across a raster of change in P. australis area between 2005 and 2019, where unequal inclusions probabilities were applied to representatively assign sites across the change in area gradient (Fig. S1). A total of 53 sites were allocated using the spatial balanced design. A further 10 sites were haphazardly incorporated into the sampling design that were on non-vegetated sediments where P. australis did not occur in either 2005 or 2019. The total number of deployments was 33 over P. australis and 30 over non-vegetated sediment. Survey sites were between depths of 1 m and 8 m, which corresponded with the depth range at which most P. australis occurs throughout the estuary.
At each site, estuarine fish assemblages were surveyed using baited remote underwater stereo-video systems (stereo-BRUVs; Langlois et al., 2020). Stereo-BRUVs were baited with fresh ~200 g of crushed pilchards (Sardinops sagax) and deployed on the seafloor for 20 min to ensure a 15 min sample. A 15 min sample and 200 g of bait was used to survey fishes in close proximity by limiting the dispersion of the bait plume (Watson et al., 2005). Sampling was done in February 2022. Before the sampling campaign, stereo-BRUVs were calibrated using the software CAL (SeaGIS, seagis.com.au) to accurately measure fish lengths and to estimate water clarity (Langlois et al., 2020). Videos were annotated using EventMeasure software (SeaGIS, seagis.com.au) and the relative abundance (MaxN) of each species was calculated. MaxN was the maximum number of individuals in any frame at any point in time (Parker and DeMartini, 1995). To calculate the relative abundance of sparids, the MaxN of each species of sparid observed (Acanthopagrus australis, Chrysophrys auratus and Rhabdosargus sarba) was summed per deployment. At the time of MaxN for each species of sparid, the fork lengths of individuals were measured. For each deployment, water clarity was estimated by making a 3D point measurement of the furthest identifiable object in the field of view using EventMeasure (Rees et al., 2021).
2.7. Analysis
We used a generalised additive model (GAM) fitted with a gaussian link to assess the relationship between total change in Posidonia australis area (2005–2019) and cumulative effects scores. The model also contained mean depth for the cell as a predictor, which was calculated from bathymetry grid (5 m resolution) derived from LiDAR (https://elevation.fsdf.org.au). Depth was included as a predictor as shallower areas may be more exposed to human activities resulting in higher stress to Posidonia australis. A GAM was chosen over a generalised linear model to identify any potential non-linear response of change in P. australis area to cumulative effects or depth. Prior to modelling the absolute values of change in P. australis extent were square root transformed to obtain a normal distribution. Notably, transformation of the response variable did not change the outcomes of the analysis (Table S2).
To test for differences in fish assemblage composition between P. australis and non-vegetated sediments, a redundancy analysis (RDA) based on Euclidian distance was used. A RDA is a constrained ordination that models the linear relationship among environmental predictors and species compositions across sites. We developed the RDA using the explanatory factors, habitat type (P. australis versus non-vegetated sediments) based on the 2019 habitat map, depth and distance to estuary mouth using the ‘Vegan’ package in R (Oksanen et al., 2013). Prior to analysis assemblage data were Hellinger transformed. A triplot was used to visualise differences in the fish assemblage between P. australis and non-vegetated sediments.
A generalised additive model (family = tweedie) was used to test the effect of distance to P. australis on the relative abundance of sparids observed on non-vegetated sediments. Distance to P. australis was calculated using the ‘near' tool in ArcGIS and values ranged from 0 m (representing the P. australis and non-vegetated boundary) to 400 m. To increase the sample size of deployments along P. australis edge, deployments up to 10 m within P. australis meadows were included in the analysis (n = 43). In the study region, P. australis is often fringed by another seagrass species - Zostera muelleri ssp. capricorni (hereafter Z. capriconi) and ten deployments on non-vegetated sediments were closer to Z. capricorni than to P. australis. To determine whether the occurrence of Z. capricorni was influencing the relationship between distance to P. australis and the abundance of sparids we reran the model including distance to seagrass (P. australis and Z. capricorni). Notably, this did not change the conclusions of the analysis (Fig. S2). Previous research has demonstrated that assemblages of small fish in Pittwater change based on distance to estuary mouth (Jelbart et al., 2007). Therefore, distance to estuary mouth was calculated using the ‘near' tool in ArcGIS for each deployment and included as a covariate in the model. Depth was also included as a covariate. Water clarity, which is likely to influence the detectability of fishes, was log transformed and included as a model offset.
To test the effect of distance to Posidonia australis on the length of sparids a generalised mixed effects model (family – tweedie) was used, which included ‘site’ as a random effect (Swadling et al., 2022). The model included the distance to estuary mouth and depth as covariates and water clarity (log transformed) as an offset. Importantly, substituting the predictor ‘distance to P. australis’ with ‘distance to seagrass’ (P. australis and Z. capricorni) did not change the conclusions of the analysis (Fig. S2). For the GAMs and GAMM, all smoothing terms were fit using a cubic regression spline (Wood, 2006), with the ‘k’ argument set to 4 to avoid over-fitting. Models were fitted using the ‘mgcv’ package (Wood and Wood, 2015) and plots created using the ‘ggplot2’ package (Wickham, 2016) in the statistical computing program ‘R' (R Core Team, 2021).
3. Results
3.1. Cumulative effects on Posidonia australis
Estimated cumulative effects scores to Posidonia australis showed spatial heterogeneity across the Pittwater estuary (Fig. 1) and ranged from 1 to 8. Cumulative effects scores were generally greatest in the upper reaches of the estuary, particularly along the southern shoreline where human development is greatest. The highest cumulative effects scores for P. australis were in the south-east arm of Pittwater where there is substantial boating infrastructure and high sediment contamination. In stark contrast, meadows along the eastern shoreline in the lower reaches of the estuary had the smallest cumulative effects scores (Fig. 1b). Low cumulative effects scores were observed for P. australis that occurred further from shore. This section of the estuary also contains the largest meadows of P. australis and had the greatest increases in extent between 2005 and 2019.
Fig. 1. a) Pittwater estuary (33.6291°S, 151.3016°E) showing the change in Posidonia australis area (m2) between 2005 and 2019 derived from high-resolution aerial imagery and b) the estimated cumulative effects scores from multiple human stressors to P. australis.
3.2. Cumulative effects and change in Posidonia australis area
The generalised additive model explained 22 % of the variation in the change in area of Posidonia australis between 2005 and 2019 (Table S2). Increasing cumulative effects were related to substantial linear reductions in P. australis area through time (edf = 2.8, F = 39.2, p < 0.0001). Areas exposed to low cumulative effects scores (< 4) were more likely to have increases in P. australis between 2005 and 2019. In contrast, areas exposed to cumulative effects scores >4 were more likely to have reductions in P. australis area through time with an increase of 1 unit above 4 corresponding to a predicted additional loss of 100 m2 over the 14 years between maps (Fig. 2 a), or 7 m2 per year. Depth was also an important predictor of change in P. australis area (edf = 2.8, F = 4.9, p = 0.002). Shallow areas of <3.5 m in depth were more likely to have reductions in P. australis between 2005 and 2019 (Fig. 2b).
Fig. 2. Predicted marginal relationship between the change in Posidonia australis area (sqrt transformed) and a) estimated cumulative effects scores from multiple human stressors and b) depth from a generalised additive model. Grey shading is ±1 standard error and grey points are the raw data overlaid.
3.3. Effects on fish assemblages
Benthic habitat had a clear effect on the fish assemblage, with a distinct composition of fishes on Posidonia australis compared to non-vegetated sediments (RDA: df = 1,59; F = 1.99; p = 0.017; Fig. 3 and Table S3). This result was driven by Helotes sexlineatus, Trachurus novaezelandiae and Trygonorrhina fasciata being more strongly associated with P. australis than with non-vegetated sediments, whereas Upeneus australiae showed the opposite pattern (Fig. 3).
Fig. 3. Redundancy analysis triplot ordination of Hellinger transformed relative abundance data constrained by habitat type and distance to estuary mouth. Grey circles represent seagrass sites while white circles are non-vegetated sediments in lefthand plot. Species labelled in righthand plot are those that contributed most to differences in fish assemblages among habitats. Note the different axes scales.
The relative abundance of sparids on non-vegetated sediments was strongly influenced by distance to Posidonia australis (edf = 1, F = 9.4, p = 0.004), being substantially more abundant closer to P. australis. Among P. australis, there were 4 times more sparids compared to 400 m away on non-vegetated sediment (Fig. 4a). Distance to estuary mouth was also a strong predictor of the relative abundance of sparids (edf = 1, F = 9.3, p = 0.004), with higher abundances in the upper reaches of the estuary (Fig. 4b). The length of sparids recorded on non-vegetated sediments was also influenced by distance to P. australis (edf = 1.3, F = 4.6, p = 0.019), where there were smaller individuals closer to P. australis. On seagrass, the predicted length of individuals was 10 cm compared to 14 cm approximately 400 m away from P. australis (Fig. 4c). Sparids were also substantially smaller in the upper reaches of the estuary compared to areas surrounding the estuary mouth (edf = 2.8, F = 6.9, p < 0.001; Fig. 4d).
Fig. 4. Generalised additive model predictions for a) the relationship between the abundance of sparids and distance to Posidonia australis, and b) distance to estuary mouth and the relationship between sparid length and c) distance to Posidonia australis, and d) distance to estuary mouth. Grey shading is ±1 standard error.
4. Discussion
Cumulative effects assessments (CEAs) are a popular approach to estimate the combined effect of multiple stressors to coastal ecosystems (Halpern and Fujita, 2013) and guide management responses (Allan et al., 2013; Anthony et al., 2013). However, few studies validate CEAs with empirical data on ecosystem condition (Stockbridge et al., 2021), due to the difficulty in defining the latter. Yet without good estimates of ecosystem condition, assessments may provide misleading conclusions. In this study, we performed a CEA for the endangered seagrass Posidonia australis. Our analysis was based on known major stressors to seagrass including foreshore development, water quality, commercial vessels, and fishing (Astles et al., 2015; Grech et al., 2012; Turschwell et al., 2021). We found a clear negative relationship between cumulative effects scores and P. australis condition (measured as change in areal extent over time), providing strong evidence for the compounding effects that multiple human stressors are having on key habitats such as seagrass and in particular this endangered seagrass population. Declines in P. australis extent were more likely to occur when the cumulative effects score was above 4, that is where the sum of the 11 standardised stressor layers was >4. As expected, our study demonstrated the influence of seagrass on fish assemblages and its importance in the seascape, with P. australis containing a distinct fish assemblage compared to adjacent non-vegetated sediments. P. australis also affected fish beyond its boundaries (or edges) as it was also an important predictor of the abundance and size of sparids on non-vegetated sediments, with smaller size classes and greater abundances near P. australis. Together, these results highlight declines in P. australis due to multiple stressors would have then flowed on to altering the fish assemblage, including species of fisheries importance.
The strong relationship between cumulative effects scores and Posidonia australis condition in the current study contrasts starkly with the only other published study to our knowledge that has sought to validate a CEA on seagrass. In the Spencer Gulf, South Australia, there was no strong relationship between seagrass condition and cumulative effects scores derived from a range of stressors (Stockbridge et al., 2021). Differing results may be due to a few reasons. First, our study used change in extent of P. australis through time rather than static condition indices (% cover, habitat structure and seagrass diversity). Although using multiple condition indices was a significant advancement on previous studies, our findings suggest that static condition indices may not be as sensitive in detecting cumulative effects compared to indices that incorporate temporal change. For example, a static approach may assume a seagrass meadow of low density and high fragmentation to be in poor condition when this may, in some cases, be an undisturbed state. Furthermore, seagrass condition indices that combine persistent and transient seagrass species may be less sensitive to impacts of cumulative effects than measures related to a temporally stable species. Second, the Pittwater estuary falls within the Sydney region, where most estuaries are characterised by large population and industrial centres resulting in the greatest proportion of cumulative risk to seagrass across Australia (McMahon et al., 2022). Therefore, differences in the types of stressors to seagrass between the two study locations may have enabled a clearer detection of impacts from cumulative effects in this study. Furthermore, the method of calculating and weighting stressors differed between the studies. Finally, Spencer Gulf is an inverse estuary (salinity greatest in the upper reaches), which may have a fundamentally different interactive effects between anthropogenic and natural stressors. In NSW, P. australis is generally restricted to well flushed, marine sections of estuaries (West et al., 1989) which typically occur near the mouth. Therefore, in the upper reaches of NSW estuaries (i.e. towards the range limit of the species) it is possible that impacts of anthropogenic stressors may be magnified. As we examined the relationship between P. australis condition and cumulative effects across the entire Pittwater estuary, our assessment may be more sensitive compared to the one conducted in Spencer Gulf, which surveyed a subset of the Gulf (Stockbridge et al., 2021). Notably, the Pittwater estuary is substantially smaller in area compared to the Spencer Gulf, making an estuary wide assessment feasible.
Unsurprisingly, mapping estimated cumulative effects scores revealed high spatial heterogeneity in the distribution of multiple anthropogenic stressors throughout Pittwater estuary. The greatest cumulative effects scores were observed in the south-east corner, which has a high density of boating infrastructure and moored/berthed vessels, and poor water quality due to the catchment containing urban and industrial land-use (Birch et al., 2012). Generally, there were higher cumulative effects scores in the upper reaches of the estuary and closer to shore. The later finding is expected given many human activities are concentrated along shorelines and in shallow depths. Importantly, the extensive P. australis meadow at the mouth of the estuary and in Careel Bay had some of the lowest predicted cumulative effects scores across Pittwater. This result is due in part to these regions having meadows that extend far beyond the shoreline, rather than fringing beds as observed in other parts of the estuary.
As generally observed, Posidonia australis contained a distinct assemblage of fishes compared to adjacent non-vegetated sediments. These findings support previous research in temperate coastal ecosystems that have observed different assemblages between these two habitats (Connolly, 1994; Davis et al., 2016; Ferrell and Bell, 1991; Guidetti, 2000; Rees et al., 2018). Differences in the assemblage were primarily driven by higher abundances of Helotes sexlineatus among P. australis. Interestingly, assemblage dissimilarity between the habitats was not as pronounced as observed in previous studies, which is most likely due to different sampling methods. As we used baited cameras to sample the fish assemblage, we were more likely to attract larger fish from broader spatial extents compared to methods such as underwater visual census and seine nets, which sample smaller cryptic species at finer spatial scales (Harvey et al., 2007; Unsworth et al., 2014). For example, previous research using seine nets to survey seagrass fishes in Pittwater have recorded numerous species from Gobiidae and Syngnathidae (Bell and Pollard, 1989; Jelbart et al., 2007), which were absent in our study. Nevertheless, our results alongside fish data collected using seine nets and unbaited cameras in Pittwater demonstrates the importance of seagrass to support fish biodiversity (Ferretto et al., 2023). Consequently, historic declines in P. australis due to multiple human stressors, and the clear importance of this habitat to estuarine fishes, indicates that these pressures would have significantly altered fish biodiversity in Pittwater.
A key knowledge gap in CEAs is its use in managing ecosystem services (Halpern and Fujita, 2013). As seagrass provides nursery habitat for a variety recreationally and commercially important fishes (Unsworth et al., 2019b), we assessed flow-on effects of changes in P. australis area to the abundance of sparids (Acanthopagrus australis, Chrysophrys auratus and Rhabdosargus sarba). On non-vegetated sediments, we found higher abundances of sparids and generally smaller individuals closer to the P. australis. These findings support previous research highlighting the significance of seagrass edge as habitat for a range of fisheries species by providing food and shelter for individuals (Connolly and Hindell, 2006; Jelbart et al., 2006; Smith et al., 2011). Previous research has also shown seagrass and non-vegetated sediments adjacent to seagrass (within 10 m) to contain higher abundances of juvenile Acanthopagrus australis and Rhabdosargus sarba compared to distant non-vegetated sediments, highlighting the importance of these habitats for the recruitment dynamics of these species (Ferrell and Bell, 1991). Therefore, it is unsurprising that elevated abundances of smaller individuals were observed nearby P. australis compared to more open non-vegetated sediment habitats. Distance to estuary mouth was a strong predictor of the abundance and size of sparids, with greater abundances of smaller individuals in the upper reaches of the estuary. Concerningly, the upper reaches are exposed to the greatest intensity of multiple stressors and have suffered the greatest declines in P. australis. Therefore, these areas may have experienced substantial change in the abundance of sparids through time. As Acanthopagrus australis, Chrysophrys auratus and Rhabdosargus sarba are among the most valued coastal species for the NSW recreational and commercial fishery, our results highlight that the loss of P. australis due to multiple stressors may be reducing fisheries production and severely compromising associated social and economic benefits. Given the ontogenetic movement of these species from estuaries to open coast reefs, impacts of seagrass loss may be affecting the abundance of sparids at spatial scales beyond the estuary (Gillanders et al., 2002; Rees et al., 2021; Swadling et al., 2022).
Our CEA and map have several applications to the management and conservation of P. australis and seagrass associated fishes in Pittwater. Our results suggest a higher likelihood of P. australis declines when exposed to cumulative effects scores >4. Therefore, in areas where cumulative effects scores are reaching this threshold, managers should weigh up the risks of adding additional stressors in an area against the benefits of the proposal. Furthermore, areas where scores are above four could be the first areas to target for management actions aimed at reducing stressors. For example, improvements in water quality or replacing boating infrastructure with eco-engineered solutions designed to limit impacts to habitat will help alleviate effects of multiple stressors (Broad et al., 2020; Cullen-Unsworth and Unsworth, 2016; Demers et al., 2013). A reduction in cumulative effects will likely enable natural recolonisation of early colonising species such as Zostera and Halophila, however, natural recovery of P. australis is slow (~8–26 cm per year) following disturbance (Cambridge et al., 2002; Meehan and West, 2000). Restoration techniques may help facilitate regrowth of P. australis, with recent examples of replanting naturally detached rhizomes showing early signs of success (Ferretto et al., 2021). This study could help target restoration work in areas where P. australis has been lost, provided management intervention has effectively reduced the current stressors. Our cumulative effects map of P. australis is publicly available (https://doi.org/10.6084/m9.figshare.22980488), which will provide a valuable resource to manage P. australis in Pittwater.
Further research will strengthen our understanding of multiple stressor impacts to seagrass and fish assemblages. Like most CEAs, our approach assumed an additive model (Korpinen and Andersen, 2016) where stressor impacts were simply summed following Halpern et al., (2008). However, a meta-analysis by Stockbridge et al. (2020), has shown that over 45 % of stressor interactions for seagrass were non additive suggesting more complex models including stressor interactions are necessary to accurately capture cumulative effects of multiple stressors (Rullens et al., 2022; Teichart et al., 2016; Turschwell et al., 2022). Our impact weights of each stressor were based on a qualitative ecological risk assessment (Astles, 2015) and assigned a value of 1, and it is possible that different weightings may have improved the fit of our cumulative effects model. For example, recent research examining the relative importance of a subset of stressors included in the current study in relation to seagrass fragmentation indicated that moorings are more likely a higher risk stressor than jetties, pontoons and aquaculture (Swadling et al., 2023). A better understanding of the different relative importance of stressors in different parts of the estuary may help to explain results for some areas where cumulative effects scores were > 4 and yet gains (rather than loses) of P. australis were identified. Future research could use a data-driven approach to determine the most appropriate weightings of each stressor and important stressor interactions by using a full subsets model selection approach (Rullens et al., 2022). This approach would also help identify the most important stressor(s) to ecosystem condition, which could be used to prioritise management actions (Brown et al., 2013).
It is also important to note that we assessed indirect effects of multiple stressors to estuarine fishes by examining changes in the condition of P. australis – a key fish habitat. Future research should strive to incorporate the direct effects of multiple stressors (e.g. foreshore development increasing shade and artificial structure) to estuarine fishes while also accounting for indirect effects such as habitat degradation. Further research is also required to test the transferability of our cumulative effects model to other estuaries and seagrass species. For instance, studies should explore the applicability of cumulative effects model on seagrass species with enduring meadows, slow growth and high conservation value in other regions around the world, such as Posidonia oceanica, Enhalus acoroides and Thalassia testudinum. If our cumulative effects model has high transferability, our findings may be used to guide management responses in different estuaries and species. Of course, there may be additional stressors or activities, which if included could improve model fit. Care needs to be taken, however, to only include stressors for which there are good data on spatial variability, have plausible or known causal links with seagrass condition, and that are relevant to the time scales of the study. Incorporating impacts of climate change (e.g. increased severity of storms that can erode or smother seagrass meadows) may be useful for future studies, but the effects of these stressors will likely vary with depth and position in the estuary. Due to a lack of data on wave and storm exposure across the entire estuary, these types of climate change stressors could not be captured in our assessment.
5. Conclusions
In conclusion, our results support the use of CEAs for assessing and managing impacts of multiple human stressors as we found a strong negative correlation between estimated cumulative effects scores and P. australis condition. To our knowledge this is the first study to validate a cumulative effects analysis for seagrass. A key finding was a cumulative effects threshold for P. australis, where cover increased through time in areas where cumulative effects scores were below 4, while declines were observed in areas where cumulative effects scores were >4. P. australis contained a distinct assemblage of fishes and areas near P. australis harboured a higher abundance of sparids, which are a recreationally and commercially important family. Therefore, we demonstrate that the change in P. australis area is likely to have impacted fish biodiversity and fisheries production within the estuary through time. These findings help fill another knowledge gap on the use of CEAs for estimating impacts of multiple stressors to ecosystem services. Finally, our results provide managers with evidence to guide their decision-making surrounding the management of P. australis and estuarine fishes in Pittwater.
CRediT authorship contribution statement
Matthew J. Rees: Conceptualization, Data curation, Formal analysis, Investigation, Methodology, Project administration, Writing – original draft, Writing – review & editing. Nathan A. Knott: Conceptualization, Data curation, Investigation, Methodology, Project administration, Resources, Supervision, Writing – original draft, Writing – review & editing. Karen L. Astles: Conceptualization, Data curation, Investigation, Writing – review & editing. Daniel S. Swadling: Data curation, Investigation, Writing – review & editing. Greg J. West: Data curation, Investigation, Writing – review & editing. Adrian M. Ferguson: Data curation, Investigation, Writing – review & editing. Jason Delamont: Data curation, Investigation, Writing – review & editing. Peter T. Gibson: Data curation, Investigation, Writing – review & editing. Joseph Neilson: Data curation, Investigation, Resources, Writing – review & editing. Gavin F. Birch: Data curation, Investigation, Writing – review & editing. Tim M. Glasby: Conceptualization, Data curation, Investigation, Methodology, Project administration, Resources, Supervision, Writing – original draft, Writing – review & editing.
Declaration of competing interest
The authors declare that they have no known competing financial interests or personal relationships that could have appeared to influence the work reported in this paper.
Acknowledgements
We acknowledge the Traditional Custodians of the land and waters of where this research was completed, and pay our respect to Elders past, present and emerging. We acknowledge that this research was completed on Country, which always was and always will be Aboriginal land. This research was funded by the NSW Government under the Marine Estate Management Strategy. The ten-year Strategy was developed by the NSW Marine Estate Management Authority to coordinate the management of the marine estate. We thank Roger Laird for assistance with validating seagrass maps, Dr. Tom Davis and Dr. Rachel Przeslawski for reviewing the manuscript and NSW Fisheries managers; Marcus Riches, Sarah Conacher, Carla Ganassin, Scott Carter and Josie Hollywood for their help developing the project.
Matthew J. Rees, Nathan A. Knott, Karen L. Astles, Daniel S. Swadling, Greg J. West, Adrian M. Ferguson, Jason Delamont, Peter T. Gibson, Joseph Neilson, Gavin F. Birch, Tim M. Glasby, Cumulative effects of multiple stressors impact an endangered seagrass population and fish communities, Science of The Total Environment, Volume 904, December 31, 2023,166706, ISSN 0048-9697, https://doi.org/10.1016/j.scitotenv.2023.166706. (https://www.sciencedirect.com/science/article/pii/S0048969723053317)
Above: Careel Bay is a Pittwater EPA
Posidonia australis, is a species of seagrass that occurs in the southern half of Australia. It occurs in 17 estuaries along the east coast of New South Wales from Wallis Lake to Twofold Bay near the New South Wales/Victorian border. Six locations within New South Wales (Port Hacking, Botany Bay, Sydney Harbour, Pittwater, Brisbane Waters and Lake Macquarie) have suffered significant population decline and have been listed as endangered populations (NSW DPI).
Why is Posidonia australis important?
- It supports a diverse range of fauna, providing habitat, shelter and food. This includes other protected and threatened species such as the White's Seahorse, Little Penguins, Green Turtles, pipefish and seadragons.
- It also provides nursery habitat and feeding grounds for commercially and recreationally important fish species such as bream, sea mullet, leatherjacket, mullet and garfish.
- Protects water quality by filtering the water and removing and recycling nutrients.
- Stabilises sediment on the seabed.
- Improves air quality by taking carbon dioxide gas out of the water and releasing oxygen. The leaves use the carbon to grow and are eventually buried in the sand when they die. This is an example of "blue carbon" - a captive of the seabed and no longer a greenhouse gas in the atmosphere.
- Did you know that seagrasses can bury carbon at a rate 35 times faster than tropical rainforests? True.